Introduction to Building Science
Learning Objectives:
- Identify the key aspects of building science and building enclosure design necessary for resilient assemblies.
- Analyze a design’s risk of condensation, based on vapor movement in a building enclosure assembly.
- Define thermal bridging and, using recent research, develop roof system design strategies that minimize the impact of thermal bridging.
- Plan approaches that prevent changes to the specifications, which can result in lower realized building performance.
Credits:
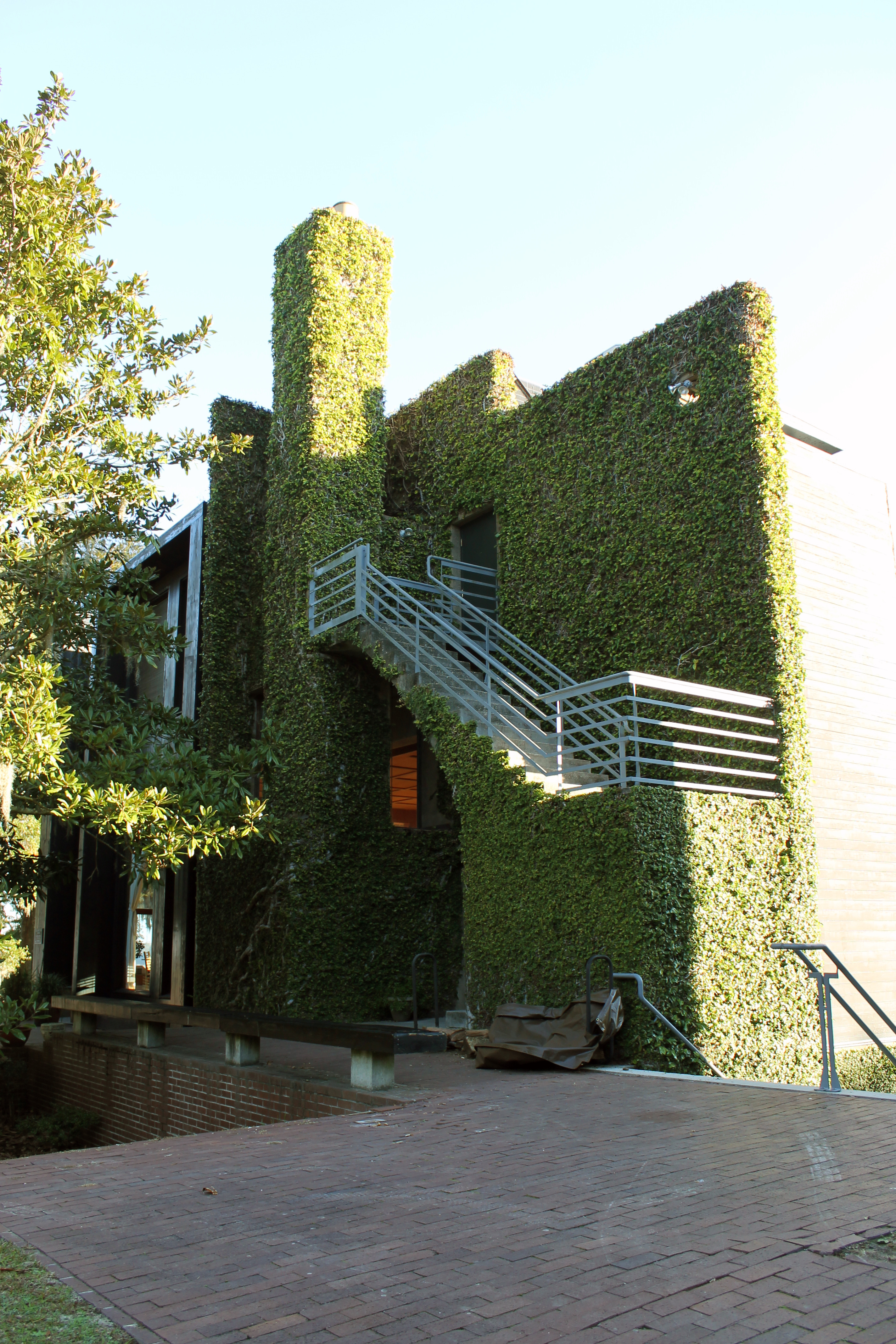
Copyright 2018 From Integrating Building Performance with Design by Elizabeth J. Grant. Reproduced by permission of Taylor and Francis Group, LLC, a division of Informa plc.
During its life, a building will face multiple challenges from its environment. Understanding the science behind design best practice can help a structure last longer, perform better, and avoid unintended consequences.
Defining Building Science
The Whole Building Design Guide defines building science as: “… a field of knowledge that draws upon physics, chemistry, engineering, architecture, and the life sciences. Understanding the physical behavior of the building as a system and how this impacts energy efficiency, durability, comfort and indoor air quality is essential to innovating high-performance buildings.”.1 In practice, building science applies what is known about physics, chemistry, and other scientific disciplines to how buildings are designed and built.
As an example, the use of building science in the design of a wall assembly may touch on concepts from material science such as the physical and chemical properties of materials like brick, insulation, and gypsum, and how those elements interact with moisture and heat. The laws of thermodynamics will be invoked to deal with heat transfer and pressure dynamics. Mechanical engineering principles are mustered to weigh the impacts of airflow and equipment that generates heat, cools air, and produces or removes moisture. Human health and biology are factors with respect to analyzing impacts of indoor air quality and the potential for mold growth within the assembly and the building as a whole. Added to all of this is a sound foundation of architecture and construction including best design practices, an understanding of the common and necessary construction techniques, and an appreciation of how the installation of materials is sequenced.
Building science is, at its core, aimed at seeing a building reach its full performance potential. In 1986, The Building Systems Integration Handbook2 gave voice to the idea of building performance, writing that “fundamentally, performance is the measurement of achievement against intention.” The authors introduced a set of criteria against which to measure the ability of a structure to match design intent based on a building’s performance. The building enclosure is not only aesthetic; its primary purpose is as an environmental separator: “It has to keep the outside out and the inside in. To do this the wall assembly has to control rain, air, vapor, and heat.”3
From the section on “Integration for Performance” by Hartkopf, Loftness, and Mill, The American Institute of Architects (AIA) defined six building performance mandates: spatial performance, thermal performance, air quality, acoustical performance, visual performance, and building integrity. Four of the six mandates can be classified under the heading of building science: thermal performance, air quality, acoustical performance and building integrity. Incorporating building science as an essential aspect of architectural design ensures that buildings are not only visually appealing but also functional.
In this introduction to building science, the principles of thermal performance, air quality, and building integrity are the focus. These elements hinge on the interaction of the materials, assemblies, and their design configurations in the building. The ultimate goal is to establish achievable performance expectations where enclosures perform as intended and they continue to perform through the project lifecycle. The durability aspects of enclosure design, including the roof assembly and how it relates to energy performance, will specifically be discussed. Rather than viewing the elements of a building as a collection of individual and unrelated components, ensuring continuity and performance of the building enclosure requires a systems perspective. Buildings have become increasingly complex systems, comprising many individual materials that must come together, stay together, and work together to avoid risks, both in the long and short term.
A Building’s Function and its Opposing Forces
It is an inescapable truth that natural forces are always at work on buildings. The fundamentals of building science have a foundation in the Second Law of Thermodynamics:
- Heat flows from warm to cold;
- Moisture flows from warm to cold;
- Moisture flows from wet to dry; and
- Air flows from areas of higher pressures to ones of lower pressures;
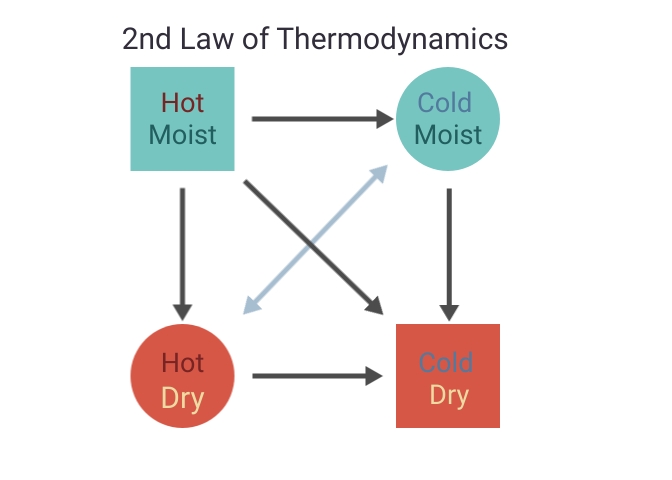
Figure courtesy of GAF|Siplast
Diagram of how the law of thermodynamics drives interaction between moist, dry, hot, and cold air.
A bonus fundamental property is that gravity always acts down. The Second Law states that energy, air, and moisture are exchanged between two systems until a state of equilibrium or balance is achieved. However, that is not necessarily the goal for a building enclosure. For example, it is not ideal to have both the interior and exterior environment hot and humid during a Florida summer. Keeping the building performing as designed often means controlling these forces. This control comes by way of control layers.
“Control layer” is the term used for the parts of the building enclosure that are designed to resist, regulate, or prevent external elements from moving uncontrolled through a structure. There are four main control layers in a building: they address liquid water, air, heat, and water vapor. Whether the control layer completely stops the movement of the elements or slows down the movement depends on the materials used. The selection of materials for each of the control layers and the location of each control layer is based on the needs of the building. In essence, the four control layers can be loosely split into two main categories: those that manage moisture and those that manage energy efficiency. Moisture management focuses on preventing liquid water, water vapor, and condensation from negatively impacting the building. Managing thermal performance or energy efficiency relies on controlling the movement of air and heat through the building enclosure.
Some control layer materials perform more than one function, and all of the control layers need to work together to achieve maximum effectiveness.
Continuity of control layers
The enclosure is better defined as a combination of control layers assembled and joined together in a system, providing a continuous boundary between conditioned and unconditioned space. Control layers are more accurately viewed as interconnected systems and assemblies instead of a single product or material. Due to the innate relationship between the building enclosure and building performance, it is critical to design for performance early in the architectural design process.
The four key control layers (water, air, thermal, and vapor) should generally be continuous across all six sides of the building enclosure. ASTM E2947 defines the term “building enclosure,” frequently used interchangeably with “building envelope,” to “refer collectively to materials, components, systems, and assemblies intended to provide shelter and environmental separation between interior and exterior, or between two or more environmentally distinct interior spaces in a building or structure.” One helpful tool to design and communicate the intent of the critical components and functions of the building enclosure is called the “pen test.” The “pen test” involves tracing each of the control layers around the building enclosure to ensure continuity. The premise is that if one has to lift one’s pen, that indicates a break in a control layer. It is relatively easy in theory, but it can get complicated at interfaces, penetrations, and transitions as is shown in Figure 3. For this reason, the pen test should be performed not only on the plans and elevations, but on the interface detail drawings where multiple materials and trades intersect. Once the control layers are defined, all materials that are used to complete the control layers in each detail should be explicitly called out and not simply shown on shop drawings as being “by others.”
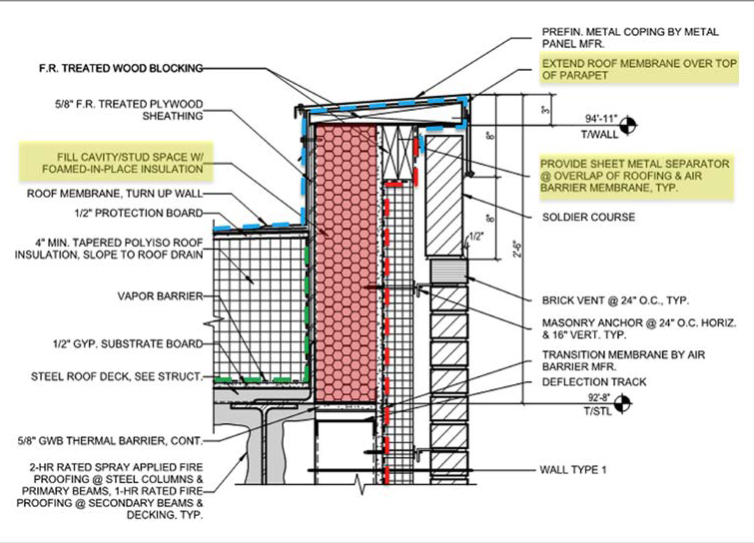
Figure courtesy of GAF/Siplast
The “pen test” is easy in theory, but it can get complicated at interfaces, penetrations, and transitions.